High-Frequency Thermoacoustic Instabilities of Auto-Ignition Flames
Motivation and Background
Thermoacoustic instability is a coupling between acoustics and heat release rate in a combustor which leads to increasing fluctuations in pressure, velocity and temperature. These oscillations can damage or even destroy the combustor. Transverse oscillations have long been an issue in rocket combustion chambers [1].
In recent years, approaches for meeting stringent emissions regulations and efficiency demands have led to high-frequency (HF) thermoacoustic oscillation problems in gas turbines used for power generation and propulsion. Typically a second stage of a sequential combustor is prone the HF thermoacoustic instabilities.
Objective
In this project we investigate HF transverse instabilities in a second stage can-type combustor where the flame is stabilized by auto-ignition (see Figure 1). To avoid such instabilities, a driving mechanism for the instability has to be understood. The goal of the project is to understand the flow-flame-acoustics interaction and to build a low-order model to make stability predictions. That will drastically decrease the design process time of a gas turbine.
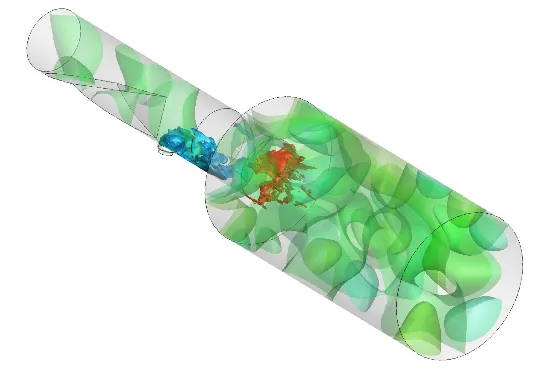
Work Approach
State of the art in thermoacoustics is the single-input single-output flame transfer function [2,3], i.e. the transfer function has only one scalar quantity as function argument and as function value. It is valid when the reaction zone is acoustically compact, i.e. the acoustic wave length is much larger than the length of the reaction zone. This is the case for low-frequency longitudinal waves. Because of the acoustic compactness, the flame can be modeled as acoustic point source and the acoustic velocity fluctuation can be assumed constant over the reaction zone. The flame transfer function FTF describes then relation between the fluctuation of the global heat release rate Q̇' (integrated over the thin reaction zone) and the velocity fluctuation at one reference point upstream of the flame
Q̇' = FTF uref',
For HF transverse instabilities the length of the reacting zone is comparable with the acoustic wave length, i.e. the flame is not acoustically compact. Thus, the flame cannot be modeled as acoustic point source any longer. Instead, the heat release rate has to be investigated locally. The cause of the local heat release rate q̇' is unknown, it can be the whole set of field quantities like velocity, pressure etc. Thus the model for the HF transverse instabilities has a general multiple-input multiple-output structure
q̇' = FTF u',
where q̇' is a vector with local heat release rate fluctuations throughout the burner domain, u' is a vector of input variables (velocity, pressure, density, species mass fractions) throughout the burner domain. The flame transfer function FTF is a matrix, i.e. the local heat release at one location is coupled to flow field in the entire burner. The problem is now very high dimensional and thus cannot be used in low order tools. We seek to reduce the dimensionality of the model. That can be done by:
- First principle modeling: a model is obtained from fundamental laws (mass, momentum and energy conservation etc.).
- Black box identification: an empirical model with no process knowledge.
- Grey box identification: empirical model with some amount of process knowledge.
This project requires knolwedge in CFD, Combustion and System Identification.
References
[1] L. Crocco. "Transverse Combustion Instability in Liquid Propellant Rocket Motors", ARS Journal, Vol. 32, No. 3 (1962), pp. 366-373.
[2] L. Crocco. "Aspects of Combustion Stability in Liquid Propellant Rocket Motors Part I: Fundamentals. Low Frequency Instability With Monopropellants", Journal of the American Rocket Society, Vol. 21, No. 6 (1951), pp. 163-178.
[3] W. Polifke. "Black-box System Identification for Reduced Order Model Construction", Annals of Nuclear Energy, Vol. 67C (0), 2014, pp. 109-128.
Acknowledgments

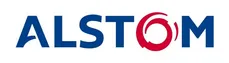
This project is funded by the Federal Ministry for Economic Affairs and Energy and by ALSTOM Power AG, whose support is gratefully acknowledged.